One evening this January, audience members packed into a lecture hall in MIT’s physics building. Undergraduates, members of the public, faculty members, and other scholars came to watch a film premiere and a panel discussion. NOVA had produced the film, “Einstein’s Quantum Riddle,” which stars entanglement. Entanglement is a relationship between quantum systems such as electrons. Measuring two entangled electrons yields two outcomes, analogous to the numbers that face upward after you roll two dice. The quantum measurements’ outcomes can exhibit correlations stronger than any measurements of any classical, or nonquantum, systems can. Which die faces point upward can share only so much correlation, even if the dice hit each other.
Dice feature in the film’s explanations of entanglement. So does a variation on the shell game, in which one hides a ball under one of three cups, shuffles the cups, and challenges viewers to guess which cup is hiding the ball. The film derives its drama from the Cosmic Bell test. Bell tests are experiments crafted to show that classical physics can’t describe entanglement. Scientists recently enhanced Bell tests using light from quasars—ancient, bright, faraway galaxies. Mix astrophysics with quantum physics, and an edgy, pulsing soundtrack follows.
The Cosmic Bell test grew from a proposal by physicists at MIT and the University of Chicago. The coauthors include David Kaiser, a historian of science and a physicist on MIT’s faculty. Dave co-organized the premiere and the panel discussion that followed. The panel featured Dave; Paola Cappellaro, an MIT quantum experimentalist; Alan Guth, an MIT cosmologist who contributed to the Bell test; Calvin Leung, an MIT PhD student who contributed; Chris Schmidt, the film’s producer; and me. Brindha Muniappan, the Director of Education and Public Programs at the MIT Museum, moderated the discussion.

I think that the other panelists were laughing with me.
Brindha asked what challenges I face when explaining quantum physics, such as on this blog. Quantum theory wears the labels “weird,” “counterintuitive,” and “bizarre” in journalism, interviews, blogs, and films. But the thorn in my communicational side reflects quantum “weirdness” less than it reflects humanity’s self-limitation: Many people believe that we can’t grasp quantum physics. They shut down before asking me to explain.
Examples include a friend and Quantum Frontiers follower who asks, year after year, for books about quantum physics. I suggest literature—much by Dave Kaiser—he reads some, and we discuss his impressions. He’s learning, he harbors enough curiosity to have maintained this routine for years, and he has technical experience as a programmer. But he’s demurred, several times, along the lines of “But…I don’t know. I don’t think I’ll ever understand it. Humans can’t understand quantum physics, can we? It’s too weird.”
Quantum physics defies many expectations sourced from classical physics. Classical physics governs how basketballs arch, how paint dries, how sunlight slants through your window, and other everyday experiences. Yet we can gain intuition about quantum physics. If we couldn’t, how could we solve problems and accomplish research? Physicists often begin solving problems by trying to guess the answer from intuition. We reason our way toward a guess by stripping away complications, constructing toy models, and telling stories. We tell stories about particles hopping from site to site on lattices, particles trapped in wells, and arrows flipping upward and downward. These stories don’t capture all of quantum physics, but they capture the essentials. After grasping the essentials, we translate them into math, check how far our guesses lie from truth, and correct our understanding. Intuition about quantum physics forms the compass that guides problem solving.
Growing able to construct, use, and mathematize such stories requires work. You won’t come to understand quantum theory by watching NOVA films, though films can prime you for study. You can gain a facility with quantum theory through classes, problem sets, testing, research, seminars, and further processing. You might not have the time or inclination to. Even if you have, you might not come to understand why quantum theory describes our universe: Science can’t necessarily answer all “why” questions. But you can grasp what quantum theory implies about our universe.
People grasp physics arguably more exotic than quantum theory, without exciting the disbelief excited by a grasp of quantum theory. Consider the Voyager spacecraft launched in 1977. Voyager has survived solar winds and -452º F weather, imaged planets, and entered interstellar space. Classical physics—the physics of how basketballs arch—describes much of Voyager’s experience. But even if you’ve shot baskets, how much intuition do you have about interstellar space? I know physicists who claim to have more intuition about quantum physics than about much classical. When astrophysicists discuss Voyager and interstellar space, moreover, listeners don’t fret that comprehension lies beyond them. No one need fret when quantum physicists discuss the electrons in us.
Fretting might not occur to future generations: Outreach teams are introducing kids to quantum physics through games and videos. Caltech’s Institute for Quantum Information and Matter has partnered with Google to produce QCraft, a quantum variation on Minecraft, and with the University of Southern California on quantum chess. In 2017, the American Physical Society’s largest annual conference featured a session called “Gamification and other Novel Approaches in Quantum Physics Outreach.” Such outreach exposes kids to quantum terminology and concepts early. Quantum theory becomes a playground to explore, rather than a source of intimidation. Players will grow up primed to think about quantum-mechanics courses not “Will my grade-point average survive this semester?” but “Ah, so this is the math under the hood of entanglement.”
Sociology restricts people to thinking quantum physics weird. But quantum theory defies classical expectations less than it could. Measurement outcomes could share correlations stronger than the correlations sourced by entanglement. How strong could the correlations grow? How else could physics depart farther from classical physics than quantum physics does? Imagine the worlds governed by all possible types of physics, called “generalized probabilistic theories” (GPTs). GPTs form a landscape in which quantum theory constitutes an island, on which classical physics constitutes a hill. Compared with the landscape’s outskirts, our quantum world looks tame.
GPTs fall under the research category of quantum foundations. Quantum foundations concerns why the math that describes quantum systems describes quantum systems, reformulations of quantum theory, how quantum theory differs from classical mechanics, how quantum theory could deviate but doesn’t, and what happens during measurements of quantum systems. Though questions about quantum foundations remain, they don’t block us from intuiting about quantum theory. A stable owner can sense when a horse has colic despite lacking a veterinary degree.
Moreover, quantum-foundations research has advanced over the past few decades. Collaborations and tools have helped: Theorists have been partnering with experimentalists, such as on the Cosmic Bell test and on studies of measurement. Information theory has engendered mathematical tools for quantifying entanglement and other quantum phenomena. Information theory has also firmed up an approach called “operationalism.” Operationalists emphasize preparation procedures, evolutions, and measurements. Focusing on actions and data concretizes arguments and facilitates comparisons with experiments. As quantum-foundations research has advanced, so have quantum information theory, quantum experiments, quantum technologies, and interdisciplinary cross-pollination. Twentieth-century quantum physicists didn’t imagine the community, perspectives, and knowledge that we’ve accrued. So don’t adopt 20th-century pessimism about understanding quantum theory. Einstein grasped much, but today’s scientific community grasps more. Richard Feynman said, “I think I can safely say that nobody understands quantum mechanics.” Feynman helped spur the quantum-information revolution; he died before its adolescence. Besides, Feynman understood plenty about quantum theory. Intuition jumps off the pages of his lecture notes and speeches.
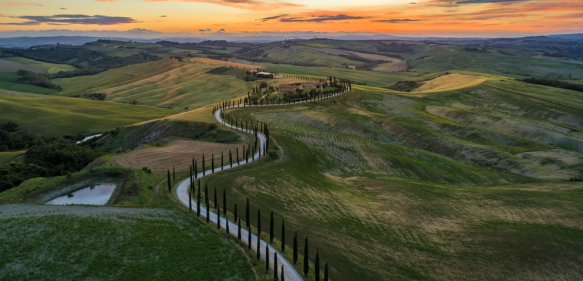
Landscape beyond quantum theory
I’ve swum in oceans and lakes, studied how the moon generates tides, and canoed. But piloting a steamboat along the Mississippi would baffle me. I could learn, given time, instruction, and practice; so can you learn quantum theory. Don’t let “weirdness,” “bizarreness,” or “counterintuitiveness” intimidate you. Humans can intuit quantum physics.
On string theory, lacking observational data, one intuits based in no small part on the beauty of its mathematics. In strong contrast, on quantum physics, one has had no choice, since the days of Planck and Einstein, but to intuit based solely on actual observations.
The weirdness of quantum physics stems from frustrated expectations when observations disagree with the biases built into our classical physics based experience. Bearing that in mind may help guide our intuition to better understand quantum reality?
Classical physics is inadequate in fully explaining quantum phenomena, nor astronomical ones between or among galaxies. Could it be plausible that we need three different sets of physics laws?
On Mon, Jan 28, 2019 at 12:25 PM Quantum Frontiers wrote:
> Nicole Yunger Halpern posted: “One evening this January, audience members > packed into a lecture hall in MIT’s physics building. Undergraduates, > members of the public, faculty members, and other scholars came to watch a > film premiere and a panel discussion. NOVA had produced the film, “” >
Certainly possible three sets of physical laws are needed. Occam’s razor would discourage.
In the opposite direction, in Nicole’s “Theoretical physics has not gone to the dogs”, a comment made on November 28 (12:06 am) happens to suggest a possible scheme relying on only one set of laws, based on a thought experiment that in theory could be performed.
Please note: Occam’s razor is not a physical theory, it is merely a philosophy. Who is to say that the solution nature uses is the simplest or most elegant? That assumption is merely a prejudice toward what is most convenient for humans studying nature. In particular, who says there has to be a “theory of everything”? It would be nice (for human students of nature) if there was one, but nature did not have this as an objective when the universe was born. All human theories are merely mathematical approximations of natural phenomenology. We can hope to get asymptotically close to any particular outcome, but the most compact exact computation of the outcome of any natural process is the process itself…a program as large as the universe.
Occam is not a theory nor any rigid philosophical dictum. It is a rough tool to encourage picking the simplest solution among many equally workable ones. My comment stands without needing to mention Occam’s razor at the beginning.
Slowpez is of course correct to ask “Who is to say that the solution nature uses is the simplest …?”. It just happens that if quantum reality is indeed “nonphysical” (lacking an existing word to describe something that is not the physical reality assumed by classical mechanics) [https://quantumfrontiers.com/2018/11/25/theoretical-physics-has-not-gone-to-the-dogs/comment-page-1/#comment-117520], it is indeed quite simple to describe nature by having classical physical reality “emerge” from quantum nonphysical reality via entanglement [https://www.preposterousuniverse.com/blog/2018/01/17/beyond-falsifiability/comment-page-2/#comment-7295910552604315312].
That this simple description of nature may make sense is supported in part both by its ability to explain Heisenberg uncertainty and by its simpler account, than the one given by the original paper, of why a black hole appears to turn into a white hole, not at the singularity, but at the event horizon [https://quantumfrontiers.com/2019/01/27/humans-can-intuit-quantum-physics/#comment-123863].
Again echoing Slowpez, despite housing classical and quantum under one roof, the above scheme lays no claim to be either “a theory of everything” nor “the most elegant”. It is what it is. It appears to simplify explanations regarding quantum uncertainty and the recent loop quantum gravity account of black-to-white hole transformation. These are but two examples.
“Quantum foundations concerns why the math that describes quantum systems describes quantum systems, reformulations of quantum theory, how quantum theory differs from classical mechanics,…”
Primarily of course it differs from classical mechanics by being probability theory rather than physics (mechanics) theory. 🙂
“Mechanics theory” is merely an approximation of “probability theory” which happens to come arbitrarily close to observable measurements at metric scales that are accessible to human senses.
The greatest stumbling block in Quatum Physics is “measurement”, the moment you measure you are classical. Lost in measurement(math) we miss the beauty. But physics is the science of measurement, so, how do we reconcile. We find it difficult to comprehend Quantum mechanics because it is so comprehensive, but we look at it in isolated chunks and then relate and connect those chunks just as is our wont with classical physics. We abstract (draw away from the whole) because thought abstracts, it is the function of thought to abstract, then we relate and connect these abstractions and make sense through this (neural) network of abstractions. But Sirs! The moment you abstract you are out of the quantum world. We abstract to gauge, to measure. Who is it that measures? The observer. Where is the observer? Relativity is as far as we have got about the observer and measurement. Thus far the observer is separate from the observed; there is “the observer”, “the observed or the measured” and “the act of measurement”; the observer himself does not influence the measurement. Different positions of the observer give different observations or measurements. Let us take the example of dopplers effect of sound. The observer on the railway platform observes waxing and waning of sound, but the same observer hears a monotonous sound when inside the train. Here, the observer is the same, but the frames of reference are different; different positions (frames of reference) of the observer give different measurements or observations. The observer himself does not interfere with the observation because he is an objective, physical observer. Thus far relativity. Now, let us look at the subjective observer. Let us the take the fly and the human as examples. The fly finds rotten stuff attractive and the human finds it offensive. But where is the offense? Is the rotten stuff rotten? The fly is biologically programmed to find the rotten stuff attractive just as the human is programmed to find it offensive. If we swap the fly’s program for the human program, then the human finds the rotten stuff attractive. The swap betrays the underlying program. So, where is the offense? It is not in the rotten stuff but in the program. The program makes the stuff offensive or attractive, does it not? We see that “the program” is the “the observer”, then what is “the observed”? It is not the stuff that is the observed, rather it is “the offense” or “the attraction” that is “the observed”. Therefore, the offense or the attraction is the virtue of the program and not the stuff, right. The program or the observer influences the observation of odor; there can be no offense or attraction (observed) without the observer or the program. Therefore “the observer” is “the observed”. In Quantum physics the observer is the act of measurement and the very act of measurement influences the observation. The observer as measurement influences the outcome. But the moment we measure we are out of the Quatum world.
Jiddu Krishnamurti is a great source.
You have got to think outside the box a particle is not a point but think of points as a location on a flexible surface Twin vortex theory you tube Anthony Davis 1949 2009 what a brilliant mind and great scienist
Picking up on thoughts expressed in the preceding comments, one out-of-the-box thinking (comment on January 29), could be physics based on not two, classical and quantum (comment on January 28), not three (comment on January 27), but one set of laws. For that to work, the incongruity between the two existing sets, classical and quantum, has to be rectified. How?
In Nicole’s “Theoretical physics has not gone to the dogs”, a thought experiment was mentioned. It is based on a modification of the standard double-slit experiment. In it, the detection screen in a laboratory on Earth is simply extended to cover the width of the observable universe (https://quantumfrontiers.com/2018/11/25/theoretical-physics-has-not-gone-to-the-dogs/comment-page-1/#comment-117520).
In so doing, the laws of both classical physics and quantum physics are brought to bear in one experiment, on one particle in space, at one moment in time, that of measurement. The upshot is the surprising conclusion that before measurement, a particle cannot belong to the physical reality assumed in classical physics, without exceeding the speed of light imposed by the same classical physics. Simply, quantum reality cannot be physical.
We have long known classical and quantum physics do not get along. By forcing them together, the thought experiment tells us exactly why that is the case. Namely, we have been incorrect in assuming, overtly or implied, as Einstein and physics have done for over a century, that quantum reality is, like classical reality, physical. By the way, that actually brings us back to the first comment on January 27.
Armed with this conclusion from the thought experiment, we have an opportunity to reduce two sets of laws in physics into one. How?
One can start by asking: which set of laws, classical or quantum, is more fundamental? Physical reality becomes undefined when faced with infinities (such as at a black hole). But “nonphysical” reality is free from such a liability. Citing this criterion, one could imagine that quantum reality might be more fundamental (https://www.preposterousuniverse.com/blog/2018/01/17/beyond-falsifiability/comment-page-2/#comment-7295910552604315312).
If the above holds water, one could try using one set of laws, that of a nonphysical quantum reality, to describe the universe.
One indication that it might work is to take seriously the idea that classical reality might emerge, via entanglement for instance, from quantum reality. This was mentioned in Sean Carroll’s February, 2018 “Why is there something rather than nothing?” (https://www.preposterousuniverse.com/blog/2018/02/08/why-is-there-something-rather-than-nothing-2/comment-page-5/#comment-7295910552604315547).
This scheme to unify of classical and quantum sounds too good to be true. Someone please point out why.
One way to make intuiting more productive is, as noted in another comment, to think out of the box. To that end, it would be helpful if one has an idea of the kind of box one is in. And also how this box prevents one from understanding quantum reality.
In a July, 2018 podcast presented by Sean Carroll in conversation with Carlo Rovelli (https://www.preposterousuniverse.com/podcast/2018/07/10/episode-2-carlo-rovelli-on-quantum-mechanics-spacetime-and-reality/) is said, “I can’t imagine being a scientist and not be a realist about reality”. We implicitly consider that reality to be physical. Einstein’s 1935 paper revealed as much: “Can the quantum mechanical description of physical reality be considered complete?” The words “physical reality” are right there in the very title.
If even Einstein, who worked tirelessly to decipher quantum reality all his life held such a firm belief that reality has to be physical, what chance do we have of breaking out of that box when intuiting quantum reality?
But then the thought experiment in “Theoretical physics has not gone to the dogs” (https://quantumfrontiers.com/2018/11/25/theoretical-physics-has-not-gone-to-the-dogs/comment-page-1/#comment-117520) showed that a particle prior to measurement (quantum reality) cannot be physical while also obeying the speed limit of light.
(For the time being, though less than ideal, that quantum reality cannot be the classical physical reality will be referred to, in the absence of accepted standard language, as “nonphysical” quantum reality.)
Paraphrasing the 1935 paper above, that thought experiment asks, “Can the classical physical description of quantum reality be considered complete?” The answer, as in 1935, is that classical mechanics is incomplete because it cannot account for quantum reality without breaking classical physics’ own speed limit of light.
The thought experiment actually confirmed one of Einstein’s predictions. At another point in the Sean Carroll podcast, Rovelli reminded us that in the year following the 1915 arrival of the general theory of relativity, Einstein predicted that one day general relativity will have to be amended to account for quantum mechanics.
We still don’t know what quantum reality is. With the thought experiment, at least we sense that it cannot be the physical reality embraced by classical physics. Specifically, classical physics has to be amended so that reality, though strictly physical in classical physics, cannot remain so when it comes to quantum physics.
That amendment might provide one way to think out of the box when intuiting quantum physics. As an example, we examine how amending classical physics could account for one essential feature of quantum mechanics: Heisenberg uncertainty.
Let us say classical physics endows us with a ruler made of titanium. It gives highly accurate readings when measuring physical objects. Yet, when used on nonphysical items such as quantum reality, the same physical ruler can be easily seen to yield uncertain readings, every single time it is used in measuring.
Using the above thinking, if a black hole is where quantum physics takes over as some conjecture, a black hole is nonphysical because quantum reality is nonphysical. In that case, it might explain why Ashtekar et al. found the black-to-white hole transition takes place not at the singularity, as naively expected, but at the event horizon (A. Ashtekar, J. Olmedo, and P. Singh, “Quantum transfiguration of Kruskal black holes,” Phys. Rev. Lett. 121, 241301 ).
The singularity and the event horizon, as black hole items, are quantum, per conjecture, and thus nonphysical. According to our titanium physical ruler analogy, both the singularity and the event horizon, as nonphysical items, will manifest quantum uncertainty. That makes saying precisely where the transition occurs, in a language suited for classical (physical) physics, less of an issue. In any case, this explanation is much simpler than citing severe spacetime distortion, as is done in the paper itself.
In short, recognizing quantum reality is not the physical reality in classical mechanics opens up a rich trove for intuition. And through focusing on black holes, such intuitions may even provide some hint of quantum gravity?
Would like to draw attention to a paper by Erik Curiel (linked below). It summarizes the latest ideas of what a black hole is, according to a wide range of physicists.
Black holes offer a fertile ground for classical and quantum to interact. Almost every word in the Curiel paper reflects the limitation imposed by a physical-only interpretation of black holes. These limitations actually give a hint at the freedom one could gain from intuiting beyond this physical-only box. One could consider, for example, the nonphysical (lacking a better existing word) quantum reality, suggested by the thought experiment, mentioned on November 28, 2018 in “Theoretical physics has not gone to the dogs”.
As an example, on p. 31 of the paper one finds “No known quantum effect, nor any other known or imagined physical process, can cause spacetime simply to stop evolving and vanish, as it were, once matter crosses its Schwartzschild radius. Perhaps nothing inside a horizon can communicate with the outside, but it does not mean it is not part of the world. As such, the mettle of physics demands that we try to understand it”.
If one is willing to think outside the physical-only box, one might not be so focused only on spacetime crossing the Schwartzscild radius, advocated first by Robert Oppenheimer and then by John Wheeler and others. Instead, one could actually cite Einstein himself, who in 1939 (“On a stationary system with spherical symmetry consisting of many gravitating masses”, Annals of Mathematics, Vol. 40, No. 4, 922.) stated that “a clock kept at [the Schwartzschild radius] would run at the rate zero”.
Why can we take Einstein’s 1939 words more seriously now? Armed with a nonphysical quantum reality inside a black hole replacing classical reality, spacetime especially, once inside the Schwartzschild radius, contrary to what Curiel wrote above, time could (along with physical spacetime), literally stop.
Lest one be too cavalier with this nonphysical remedy, there remains the issue of the gravitational effects of black holes. On that, Wheeler did use “attraction of disembodied mass” (see John Preskill’s “Entanglement = Wormholes” https://quantumfrontiers.com/2013/06/07/entanglement-wormholes/comment-page-2/#comment-82042). Similarly, in the Curiel paper, ‘t Hooft said “A black hole is … gravity without matter” (p. 32). By the way, this similar characterization of black holes and dark matter is interesting.
“The many definitions of a black hole”, Nature Astronomy Vol 3, pp. 27-34, January, 2019
Hi. I like this movies. It supports my own conclusions that I made in my experimental research which rethinks the current physics. This world may be based on the quantum entanglement. For example, I suppose the current physics cannot explain how astronauts can so easily move subjects (masses) in International Space Station (ISS) if even a small mass (1 kg) has the colossal moment of Inertia about 45,846,441,000,000 kg m^2 aboard ISS 🙂
Yes, human beings can feel it thru spiritualism. It’s ‘weird’ because we can’t explain or prove what we experience; similar to ‘God’ or ‘consciousness’. We need to experience lot instead of gaining more knowledge of it.